
In our previous post we covered the details of the mechanisms coronavirus uses to infect and replicate in our bodies. So, now, at last, let us see why Biology is the solution by looking at the different options we already have in hand or under testing to start taking control of this situation.
One of the biggest problems with viruses is that we actually do not know how to kill them at all once they are inside us. All we do is weakening them as much as possible to buy time for our own immune system to generate the right tools (mainly antibodies) to eliminate them.
The strategy we have taken in the past to mitigate or even eradicate viral diseases is mass vaccination and herd immunity. Nevertheless, an effective and safe vaccine will take months to develop, approve and manufacture (not a minor problem this latter), so in the meanwhile we will have to try other approaches to buy time. Many vaccines are right now under development, however, it should also be taken into account that there is currently no vaccine for any kind of coronavirus and that some of the vaccination approaches pharmaceutical companies are testing are based on molecular mechanisms with no precedent amongst approved drugs. Therefore, there is a lot of uncertainty around the feasibility of a vaccine (we have not been able to develop vaccines for many viruses yet) and in the best-case scenario, how long will it take before it is broadly available. On this end there is a very recent finding that suggests that the BCG vaccine, containing the inactivated bacterium Mycobacterium bovis, a close relative of the agents causative of tuberculosis and leprosy, may be protective against COVID-19. Promising, although results are still very preliminary (more on this later).
Unless any other approach proves effective, our option is just trying to drug the virus. Novel drugs also take time to develop, test and approve. This is the reason why pharmaceutical companies and doctors are trying to use existing, already-approved medications, in a process known as drug repurposing or drug repositioning (similar to the approach with the BCG vaccine mentioned above). At the same time, novel non-vaccine strategies are being put in place. Let us look at the different molecular alternatives we have, based on SARS-CoV2’s biology and infective cycle.
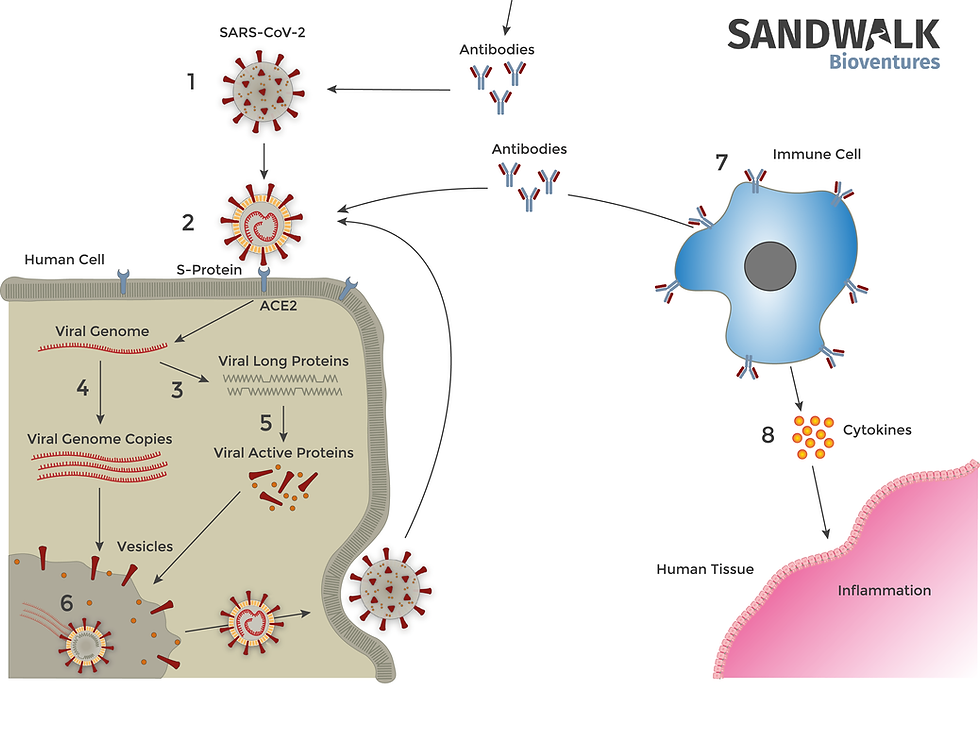
1. Killing the virus before it enters the cell
The immune system is the ultimate solution for the virus. However, generating proper defenses against it can take time, and this time may be too long for some patients which cannot survive that long with the infection, or which may be immunocompromised for different reasons.
Therefore, one of the obvious potential approaches is using pre-generated immunity by injecting antibodies against the virus in diseased individuals. This is technically known as passive immunization and was one of the strategies employed in the 2013-2016 ebola epidemic. In that case antibodies generated by cured individuals were used, and this is indeed one of the options looked at for coronavirus and actually tested successfully in small trials. Since extracting these antibodies from people’s blood is a slow process and not exempt from risks, companies have identified the crucial antibodies generated by people which successfully overcame the disease and are now manufacturing them industrially. Companies like Takeda, AbCellera Biologics/Eli Lilly, Harbour Biomed, ImmunoPrecise Antibodies and the consortium Vir Biotechnology/WuXi/Biogen are working on this strategy.
2. Preventing viral adhesion and entry
One of the crucial steps in infection is the binding of the virus to its target, which in this case, as we have seen, is ACE2. The fact that ACE2, but mainly ACE1, are involved in the regulation of blood pressure in humans initially led some to suggest that antihypertensives may be an option to block viral recognition of its ligand. However, mechanistically this is not correct. We will speak about this in our next post about COVID-19.
Focusing on the specificity of the virus for ACE2, the company APEIRON Biologics a clinical trial with its drug candidate APN01, which is a genetically engineered copy of the actual human ACE2 initially developed for lung injury and hypertension. In theory, it could trick SARS-CoV2 and make the virus bind to it instead of to the similar receptor attached to a cell, thus preventing viral entry.
3. Not letting our own cells create long viral proteins
One of the most crucial steps of the hijacking process of our cells is the generation of viral proteins by our own human or human-mitochondrial ribosomes.
There are a number of drugs, mainly antibiotics such as chloramphenicol and azithromycin, initially designed to kill bacteria, that work by inhibiting protein synthesis. Since mitochondria inside our cells are similar to bacteria (this is a very long and amazing story), these antibiotics do also attack their ribosomes and may thus be useful in fighting the virus. In fact, in a trial which results were published on March 20th, the combination of hydroxychloroquine (about which will speak later) plus the antibiotic azithromycin was significantly more effective than hydroxychloroquine alone which may point in the direction that this approach may be worth exploring.
We can also actually use lab-made RNA to physically block the viral genome so that ribosomes cannot read it. This is done with a relatively novel technique called RNA interference, by which we can create a strand of RNA complementary to the genome of the virus so that they spontaneously bind together when they are close. This prevents the binding of ribosomes and hence the production of proteins vital for the virus. The companies Alnylam Pharmaceuticals, Vir Biotechnology and Sirnaomics are working in this RNA interference approach.
4. Not letting the viral polymerase create new viral copies
This is one of the strategies being looked at more closely, as it is also a common one to fight viruses. For example, the acyclovir we take for our cold sores or the famous Hepatitis C drug Sovaldi (sofosbuvir) have this mechanism.
In order to create new copies of the genome of the virus, the viral polymerase needs building blocks (called nucleotides), the same way our own polymerases need them to create new cells for ourselves. Viral and human polymerases serve the same purpose but have many molecular differences, which also determine that the building blocks each of them need are also different (remember that our genome is made of DNA and coronavirus’ is RNA). So, if we provide the viral polymerase with building blocks specific for it but that are wrong, it will create defective copies of the virus which will not be able to infect other cells.
Gilead’s remdesivir, originally developed as an ebola treatment, has shown efficacy against a number of RNA viruses including other coronaviruses; Fujifilm’s (yes, the photo film maker) favipiravir was designed for flu, but recently showed capacity to clear SARS-CoV2 infection in four days; Biocryst’s galidesivir is a broad-sprectrum antiviral which acts against over 20 RNA viruses; and the new compound EIDD-2801 has shown early promise in vitro.
Here the above-mentioned RNA interference can also play a role. In the same way we could block the binding of ribosomes to the viral RNA, we can block the binding of the viral polymerase to the genome and its copies, thus stopping the process of viral replication. The companies Alnylam Pharmaceuticals, Vir Biotechnology and Sirnaomics are working in this RNA interference approach.
5. Not letting proteases create viral functional proteins from long viral proteins
Many current antivirals for HIV and hepatitis act through this mechanism, and proteases have been named the Achille’s heel of coronaviruses.
However promising, a combination of two antivirals with this mechanism, lopinavir and ritonavir, failed to improve clinical outcomes of COVID-19 in a recent trial. Nevertheless, the World Health Organization (WHO) recently launched a human trial to re-test the efficacy of these two agents combined. Also, Ascletis Pharma’s combinations of antivirals with this same mechanism, namely danoprevir (approved for hepatitis C) and ritonavir (approved for HIV), oseltamivir and ASC09, and Janssen’s cobicistat are being tested right now.
The problem with this strategy may reside in that not only viral, but also human proteases may serve this purpose in the infection leaving the human protease activity as an emergency backup for the virus. This is thought to be one of the reasons SARS-CoV2 can spread to so many tissues in humans, why it may be extra deadly and may also may explain the lack of efficacy of this therapeutic approach.
6. Stopping cell vesicle creation and release
Although this step may look as a lower-relevance mechanism, drugs that interfere with this process are amongst the most promising ones, as preventing the formation of loaded vesicles with new viruses effectively prevents their release and thus their spread to new cells or hosts.
That is the case of the related molecules chloroquine and hydroxychloroquine, licensed for the treatment of malaria and also rheumatic disease. After promising results in vitro and in COVID-19 patients the WHO and other parties have decided to bet on these two molecules for its large-scale clinical trials.
7. Boosting our own immune system
Trying to debilitate the virus through various mechanisms is an option always to go for, but so is trying to boost the patient’s immune system to help it defeat the infection.
The mechanism by which the BCG vaccine mentioned at the beginning seems to be working is actually by preparing our immune system to fight pathogens in general, coronavirus being of them. It is noteworthy that this same therapy is being currently used to strengthen the immune system to treat cancer. Cancer is not an infectious disease, but is something our defenses have to fight against, just like SARS-CoV2.
Interferon beta, a molecule which is part of the natural defensive system of our cells against viruses, is also hypothesized to be potentially effective. This compound, in combination with other agents cited above, is being tested for efficacy by the WHO. Other molecules like AIM ImmunoTech’s rintatolimod do also activate the immune system through more complex pathways.
A more technically sophisticated approach consists in actually introducing entire immune cells via cell therapy, like Celularity’s CYNK-001, originally designed for cancer. These cells can eliminate infected cells to stop the production of viruses and can also collaborate with other elements of the immune system to boost for instance interferon beta response.
8. Reducing excessive inflammation
Although having a properly empowered immune system is important to fight the infection, an exacerbated immune response against the virus is indeed being the cause of many deaths by coronavirus, as the tremendous inflammation in the lungs makes it very difficult to oxygen to enter our body – hence respiratory difficulties and ultimately suffocation. This is why a number of approaches, some of which may cause initial surprise, are being considered.
Additionally to antibodies to actively fight the virus, our immune cells generate a whole plethora of substances as a response to a threat, and many of them lead to inflammation. For this reason, antibodies directed not against the virus, but rather against the pro-inflammatory molecules generated by our body to stop them, are being tested to lower inflammation in the lungs. Since some inflammatory and autoimmune diseases, as well as some cancers, share mechanisms with this virus-induced inflammation, some drugs initially developed for cancer (EUSA Pharma’s siltuximab) or conditions such as rheumatoid arthritis (Regeneron/Sanofi sarilumab and Roche’s tocilizumab) are being tested for COVID-19.
Other strategies
These potential strategies above pay attention to some crucial steps in the viral replication mechanism, driven by a handful of viral proteins. However, the genome of SARS-CoV2 is amongst the largest described for RNA viruses and in fact codes for around 30 proteins, some of which could constitute potential, additional weak points.
So far we have mainly looked at the targets within the viral cycle we can use to defeat the infection. However, by also taking into account the human side of the interaction, equally required by the virus in order to replicate itself, the options broaden significantly. For instance, a recent study identified 332 different molecular interactions between SARS-CoV2 and human proteins, which yielded 66 potential human druggable targets which may be actioned by 69 existing FDA-approved drugs.
We should keep in mind that these strategies are not mutually exclusive, and in fact many trials are working with combinations of several of these agents to tackle the problem from multiple directions. Indeed, the WHO has launched four large-scale clinical trials with a) remdesivir alone; b) chloroquine and hydroxychloroquine; c) ritonavir+lopinavir and d) ritonavir+lopinavir+interferon beta.
Anyway the answer, as always in these cases, is in Biology.
References
Commenti